Stellarators are advanced magnetic confinement devices designed for plasma fusion research. Understanding and controlling impurity transport within the stellarator environment is crucial for optimizing plasma performance and maintaining the stability of the fusion process. This research project aims to make important steps towards a comprehensive simulation model to study the transport of impurities in a stellarator, with the ultimate goal of enhancing the efficiency and reliability of future fusion reactors. The primary objectives are to develop a numerical model for simulating collision-driven impurity transport in a geometry close to the complex magnetic geometry of a stellarator, to investigate the impact of various plasma parameters, magnetic configurations, and to identify and analyze potential mechanisms influencing impurity confinement in a stellarator plasma.
The high magnetic field made possible by high temperature superconductors in the Renaissance Fusion (RF) project is expected to reduce both neoclassical (collisional) and turbulent transport, which both scale like the Larmor radius. This includes the transport of energy, angular momentum, and particles. Low transport level of electrons, fuel ions and alphas is desirable, for it will improve plasma confinement and fusion heating. Low transport of He ashes and impurities, though, could pose a problem of plasma dilution. The consequences on impurity transport of the expected high density plasmas, allowed by the unusually high magnetic field, will also be explored.
Therefore, understanding and controlling impurity transport in stellarators is crucial for advancing fusion research. Due to the non axi-symmetry of such devices, collisional transport is known to play a prominent role. The anticipated outcomes of this research project include: (i) a validated numerical model for simulating impurity transport in stellarator-like, high magnetic field, high-density plasmas relevant for RF stellarator; (ii) insights into the key factors influencing impurity behavior in different stellarator configurations; (iii) guidelines for optimizing stellarator operation to minimize impurity contamination and enhance plasma performance.
The proposed work will particularly focus on neoclassical transport, which can be dominant in non axi-symmetric devices such as stellarators. As a starting point, neoclassical transport of various impurities will be analyzed when scanning three of the main dimensionless parameters in tokamaks (normalized gyro-radius, collisionality and aspect ratio) for a given three-dimensional (3D) configuration of stellarator-like magnetic equilibrium. Important deviations from tokamaks are expected, due to the greater variety of trapped particle "families" in stellarators. Last but not least, the unusually low aspect ratio of the RF stellarator will further enrich the trapped-particle physics. If time allows for, the second stage of the work could include a sensitivity scan of the main findings with respect to the spectrum of the 3D magnetic equilibrium.
To this end, the Gysela code will be used. This is a gyrokinetic code developed at CEA-IRFM, parallelized and exploited on super-computers among the most powerful in the world [Grandgirard 2016]. It is able to describe kinetic ions as well as kinetic trapped electrons. It was initially developed to describe the plasma behavior in the core of a tokamak where the magnetic configuration is 2D. Recently, it was upgraded to model toroidal variations of the magnetic field strength, and applied to study effects of ripple [Varennes 2023]. In the latter case, the magnetic configuration is in a sense “quasi-2D”, the direction of the magnetic field vector remaining 2D. In a stellarator, the magnetic configuration is fully 3D. The goal of this project is to take a step towards full-3D geometry by incorporating in the code a magnetic strength that depends on the three dimensions in space, in a way as close as possible to that of typical stellarators, while the vector itself will remain 2D. Full 3D modelling will be left for future work. The outputs of the code will be benchmarked on the very few codes capable to handle full 3D geometries, notably the local code NEO devoted to neoclassical transport [Belli 2015].
Using the Gysela code, which has the advantage of being global and flux-driven, could shed light on the following:
- Steady-state or quasi-steady-state modeling of the impurities: density and temperature profiles of lithium, tungsten, alpha and Helium ashes.
- Quantification of diffusivities and other impurity transport coefficients.
- Modeling and parametrizing their dependence upon field, density, and aspect ratio.
To further improve the understanding of impurity transport, in complement to Gysela simulations, two codes (TAPAS [Zarzoso 2022] and ASCOT [Sipilä 2021]) enabling the study of particle trajectories in a given magnetic configuration could be used to finely analyze the complex trajectories of charged particles in a complex 3D magnetic configuration.
The research will combine numerical simulations, theoretical analysis, and experimental validation:
- Numerical simulations: use the state-of-the-art plasma simulation code GYSELA to model impurity transport in stellarator-like configurations. Incorporate 3D magnetic field magnitude configurations and plasma profiles.
- Theoretical analysis: develop analytical models to gain insights into the fundamental physics governing impurity transport and identify key parameters influencing impurity behavior.
- Experimental validation: collaborate with experimental stellarator facilities to compare simulation results with observations, validating the accuracy and reliability of the developed model.
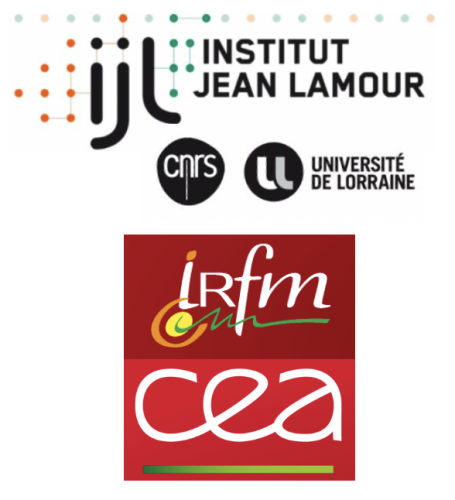